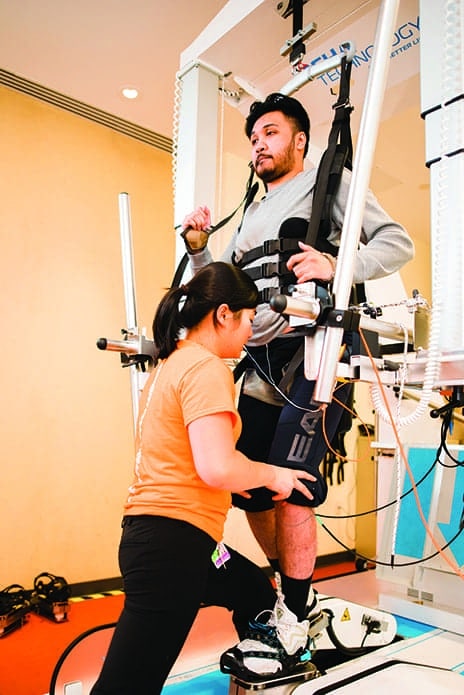
One of the advantages of this gait training system is that it uses end-effector technology to assist patients in stepping, while a therapist provides manual facilitation. (Photo by Kevin Hentz)
by Rebecca Martin, OTR/L, OTD, and Dennis Tom-Wigfield, PT, DPT
Investment in therapeutic technologies spans a continuum from elastic bands that cost a few dollars to room-sized mobility and balance systems that require construction build-outs and additional staff. Inhabiting the middle to upper range of this continuum are robotic devices and associated technology, which have become increasingly popular. Though these advanced technologies deserve a thorough cost-benefit analysis and review of competing products prior to purchase, the payoff they may provide in outcomes and efficiency can make the investment well worth the effort.
Among the facility-based technologies that have grabbed recent headlines, robot-assisted therapy is one that may be attractive to healthcare organizations. Robot-assisted therapy is an efficacious method to remediate disability associated with a wide variety of neurological disorders, most notably stroke and spinal cord injury (SCI). Intensity and repetition has been repeatedly demonstrated to be necessary for central nervous system excitation and associated motor learning.1 Massed practice, or high-volume repetition, has been shown to improve muscle strength and voluntary function.2 Robot-assisted therapy has the capacity to provide high numbers of specific movements with support or guidance as necessary, ensuring optimal conditions for motor learning and recovery of function.3 Changes can be observed in as little as 6 weeks and peak around 12 weeks of training.4
Nearly all robotic devices include some sort of computer interface, even a virtual reality component, providing the patient and therapist with real-time feedback to improve performance. Robotic devices also allow for quantitative monitoring; measuring changes in strength, range of motion, and trajectory; and illuminating patient engagement trends, time, and effort.3 As the body of literature expands and supports its use, patients are seeking clinics with these resources. Robotic technology has the potential to align patients’ interests in validated strategies with clinics’ interests in efficiency and payor-supported interventions. Clinics have an opportunity to improve patient outcomes and efficiency with which they reach those outcomes by investing in robotic devices. This investment is not trivial, however, and better understanding of the capacity and scope of different devices will help to make sure that everyone’s resources are utilized appropriately.
Assessment: Get the Complete Picture
Before it begins to investigate and trial devices, a clinic should do a careful self-assessment. Clinics should have a good understanding of their patient factors and needs: demographics, diagnoses, and payor mix. Equally important, clinics should have a good understanding of how much of their own resources—money, time, and space—they have to spend. Although money is often considered to be the limiting factor in the acquisition of technology, time and space deserve equal consideration. Nothing would be worse than investing in the perfect body weight support (BWS) gait trainer, only to find that your ceiling is too low to accommodate it. Similarly, clinics should anticipate that therapists will need time outside patient care to learn the devices and that efficiency will suffer in the early learning phase. Clinics will want to consider existing technology and therapist-driven interventions when deciding on their specific needs. Clinics would benefit from having a clear plan for acquisition and incorporation of robotic technology into existing practices. Acquiring too much technology too quickly is a sure way to reduce integration of devices and waste valuable resources.
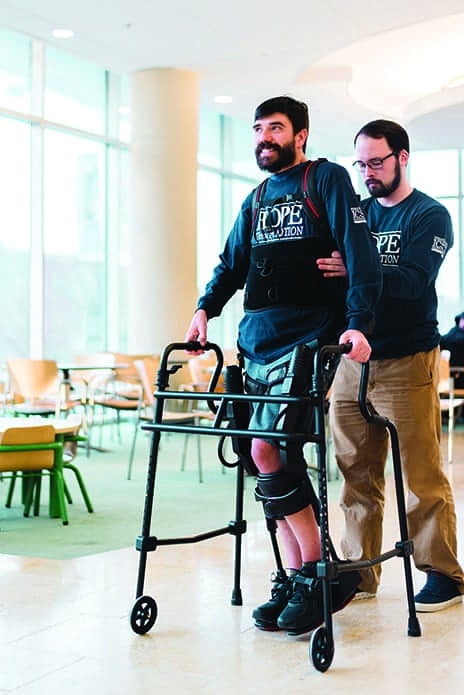
Motors in the hip and knee assist the patient in taking a step in this exoskeleton device. The therapist controls the amount of assistance, making it a good option for a wide variety of users. (Photo by Kevin Hentz)
Technology Options
There are two major categories of robotic devices that a clinic may consider: end-effector and exoskeleton-based. End-effector devices control motion of the limb through contact with the distal segments, whereas exoskeleton-based devices support the entire limb, providing support or guidance at each segment.5 End-effector devices accommodate a wider range of body morphology and offer the opportunity to combine interventions, by adding electrical stimulation or manual facilitation, but may not provide sufficient control of the limb. Exoskeleton-based devices have the capacity to provide greater support and control, but their applicability is limited by fit. FES orthoses are often also included in the realm of robotic devices, as they create conditions for high-volume repetition, offer performance feedback, and quantify changes. Each type of device has advantages and disadvantages, which deserve careful consideration by clinics interested in purchase.
End-Effector Robotic Gait Trainer
One of the investments in rehabilitation technologies made by this facility was directed at providing therapists with a BWS robotic gait trainer that utilizes end-effector mechanics. For this specific technology, patients are suspended by a harness over two mobile force plates. Using ground reaction forces, the device aims to create a near-normal gait pattern by contact only at the patient’s foot and ankle. This device has both a level stepping mode and stair climbing mode which can be utilized in passive, active-assistive, or active mode. Adjustments can be made for body weight support, step cadence, velocity, step length, step height, and ankle angle. The facility’s end-effector robotic gait trainer is indicated for patients with flaccid or active lower extremities and allows for adult and pediatric setup. The adjustability of the device and its applicability for a range of patients with a wide range of deficits make it an attractive option for many clinics. In patients with chronic stroke, end-effector robotic gait training was found to significantly improve global motor function, reduce spasticity, improve endurance with ambulation, and improve balance and coordination.6 Patients affected by Parkinson’s disease show similar improvements in gait mechanics with robotic training, over treadmill training.7 Patients with incomplete spinal cord injury demonstrate increased gait speed, endurance, lower extremity activation, and ability with over ground ambulation after robotic gait training in this device in addition to FES cycling.8
Only one skilled technician typically is required for setup and implementation of the end-effector robotic gait trainer. This system is more efficient than traditional BWS treadmill gait training devices that require three to four trained professionals. Setup in the device’s custom harnesses is quick in standing and can be easily performed on a hi-low mat table for patients unable to stand. The device is often used as a gait trainer for patients with profound weakness throughout their entire body, for patients with strong LE tone that limits use of other devices, and for patients who are part-time to full-time ambulatory. Non-ambulatory patients work on core strengthening, weight shifts, upright positioning, and massed repetition stepping while in the robotic gait trainer, whereas ambulatory patients work on gait mechanics and lower extremity strength. The device excels at providing robotic gait training for a wide variety of patients with a low efficiency requirement for the clinic. This comes with a steep price tag and a footprint and ceiling height requirements that may be prohibitive for small clinics.
The facility has also acquired an exoskeleton robotic gait trainer that allows for over-ground ambulation. This exoskeleton is designed to support itself and the patient’s weight, allowing them to experience full weight bearing while walking regardless of leg strength. Multiple modes allow the therapist to choose whether the device should facilitate movement, be passive, or actively resist patient movement. The therapist has the option to program different assistance or resistance levels to each leg, and the exoskeleton has the ability to perform squats, unilateral stepping, and side stepping. The exoskeleton robotic gait trainer is indicated for patients with a lower extremity weakness due to stroke, SCI, and most other neurological conditions. While it is appropriate for a wide range of diagnostic groups, the technology requires patients to have higher levels of function, including one upper extremity, than the device itself. The exoskeleton robotic gait trainer has demonstrated the ability to improve walking speed, increase walking distance, recover lower extremity muscle firing sequence, and decrease gait deviations.
Startup costs of the exoskeleton robotic gait trainer, in money, and in time spent training staff, are high. Individual patient setup can also be time-consuming, as each moving part of the robot needs to be custom-fit to the patient. An evaluation is performed prior to initial use of the machine to measure the patient’s hip width, upper leg length, lower leg length, lower extremity joint ROM, and arm and leg strength. As clinics consider investment in robotic devices, they should consider additional equipment which supports its application. Use of a height-adjustable hi-low mat table is crucial to ensure measurement of the leg in a sitting position that allows for more than 90 degrees of hip flexion and 90 degrees of knee flexion. Clinicians will find that a mat with hands-free adjustability and a smooth surface for transfers is most useful. Hip extension range of motion and upper leg measurements are performed lying down on the mat table as well. The exoskeleton is operated by just one therapist; however, the therapist must complete a week-long certification training program provided by the manufacturer. Clinically, the exoskeleton robotic gait trainer is indicated to teach weight shifts, to improve body awareness, to improve core strength, and to promote neuromuscular recovery with massed repetition stepping.
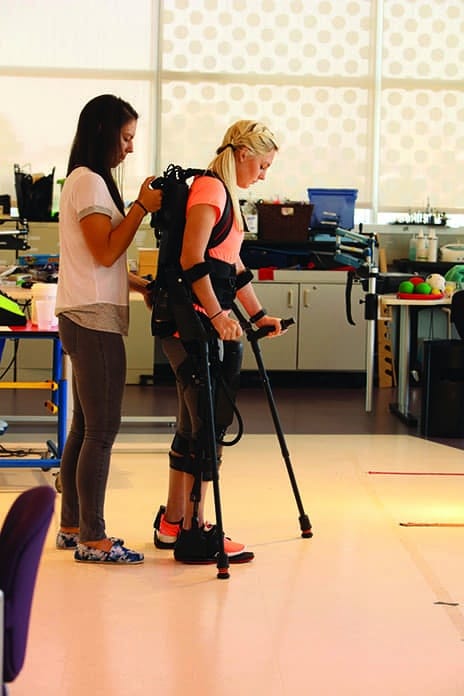
A physical therapist assists this patient in weight shifting to initiate a step in an exoskeleton. The device allows the therapist to control step height and length, while the patient initiates with a weight shift.
Functional Electrical Stimulation
To assure therapists have a variety of technologies they can call on to address a spectrum of patient needs, ICSCI has also invested in functional electrical stimulation (FES) devices designed to treat patients affected by drop foot. The facility has devices built by two different manufacturers, both of which use FES to improve toe and foot clearance during gait, and can be used therapeutically or for everyday use in the community. The devices are indicated for individuals with a wide variety of neurological injuries that affect upper motor neurons. FES devices negate the need for orthotics and provide strength training to the remaining nerve pathways and muscles. One of the facility’s devices uses “tilt sensor technology” to determine the angle of the tibia during the gait cycle. Once the angle is achieved, FES is applied to the peroneal nerve to improve toe clearance while walking. This allows patients to use the device with any shoe or sandal, as well as while barefoot.
A second FES technology at ICSCI uses a heel switch in the patient’s shoe to trigger peroneal nerve stimulation for toe clearance during gait. This system may be more consistent for different types of gait such as jogging or going up/down stairs. However, it limits the footwear a patient can use with the device. This device also has a thigh FES component to stimulate the quadriceps or hamstrings. The thigh component can help prevent buckling during stance phase of gait or help improve knee flexion during swing phase of gait. Both devices are programmable by a skilled therapist to meet the unique needs of each patient with drop foot. They can be fit to a wide range of adult patients, and take up very little clinic space. Training is important for successful application, so time investment is high initially, but devices are often covered by insurance, allowing for good carryover rates and higher efficiency within a patient bout of care.
Robot Ready
Ultimately, it is the result of their careful self-assessment, which will elucidate clinics’ need and readiness for robotic technology. Quality randomized, controlled trials are limited on the topic, so clinics will make decisions based on their available resources and needs. Robotic-assisted therapy is not a replacement for knowledgeable clinicians. At the end of the day, it is a careful assessment by a therapist, the therapeutic relationship, and evidenced-based interventions that will drive change in our patient populations, with or without a helpful robot. RM
Rebecca Martin, OTR/L, OTD, is an occupational therapist and Manager of Clinical Education and Training at the International Center for Spinal Cord Injury at Kennedy Krieger Institute in Baltimore, where she has been since 2005. She is also an assistant professor at the Johns Hopkins School of Medicine in the Department of Physical Medicine and Rehabilitation. Rebecca received her Bachelor’s degree in Occupational Therapy at Boston University in 2001 and her Doctorate from Rocky Mountain University in 2008.
Dennis Tom-Wigfield, PT, DPT, is a Physical Therapist II and part of the robotics team at the International Center for Spinal Cord Injury. He studied for his DPT at the University of Maryland, Baltimore, and began working in neuro-rehabilitation at Kennedy Krieger Institute after graduation. For more information, contact [email protected].
References
1. Behrman AL, Harkema SJ. Physical rehabilitation as an agent for recovery after spinal cord injury. Phys Med Rehabil Clin N Am. 2007;18(2):183-202.
2. Beekhuzien KS, Field-Fote EC. Massed practice versus massed practice with stimulation: Effects on upper extremity function and cortical plasticity in individuals with incomplete cervical spinal cord injury. Neurorehab and Neu Rep. 2005;19:33-45.
3. Lo AC. Clinical designs of recent robot rehabilitation trials. Am J Phys Med Rehabil. 2012; 91 (Suppl): S204-S216.
4. Lo AC, Guarino PD, Richards LG, et al. Robot-assisted therapy for long-term upper-limb impairment after stroke. New Eng J Med. 2010;362(19):1772-1783.
5. Sale P, Mazzoleni S, Lombardi V, et al. Recovery of hand function with robot-assisted therapy in acture stroke patients: a randomized-controlled trial. Intl J Rehab Res. 2014;37:236-242.
6. Mazzoleni S, Focacci A, Franceschini M, et al. Robot-assisted end-effector-based gait training in chronic stroke patients: A multicentric uncontrolled observational retrospective clinic study. NeuroRehabilitation. 2017;40(4):483-492
7. Galli M, Cimolin V, De Pandis MF, et al. Robot-assisted gait training versus treadmill training in patients with Parkinson’s disease: a kinematic evaluation with gait profile score. Funct Neurol. 2016;18:1-8.
8. Hesse S, Werner C, Bardeleben A. Electromechanical gait training with functional electrical stimulation: case studies in spinal cord injury. Spinal Cord. 2004;42:346-352.